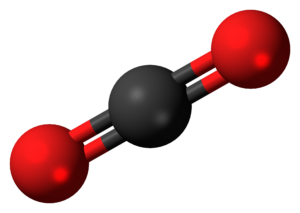
Welcome back to our “Molecule of the Month” series, where we delve into the world of atmospheric pollutants and greenhouse gases. With its minimum annual mean global concentration this month (419.7 ppm), we focus on Carbon Dioxide (CO2), a molecule that plays a pivotal role in shaping our environment, climate, and health. In this post, we will explore the sources, effects, and common techniques to monitor CO2 levels in the atmosphere.
Carbon Dioxide (CO2) is a colorless, odorless, non-flammable gas of utmost importance, with far-reaching consequences. It impacts various aspects of our world:
1. Human Health: While not directly harmful in the concentrations typically found in ambient air, excess CO2 can contribute to indoor air quality issues, affecting comfort and cognition in enclosed spaces. The Occupational Safety and Health Administration (OSHA) of the USA has established for an 8-hour work day an averaged exposure limit of 5,000 ppm (0.5% CO2 in air).
2. Climate and Environment: CO2 is the major greenhouse gas with a larger overall warming influence than all of the other greenhouse gases combined. In 2013, the increase in CO2 was estimated to be responsible for 1.82 Wm−2 of the 2.63 Wm−2 change in on Earth. The global average concentration of CO2 in the atmosphere increased since the start of the to 421 (May 2022), up from 280 ppm during the 10,000 years prior to the mid-18th century. This is an increase of 50%.
3. Ocean Acidification: When CO2 dissolves in the ocean it can react with H2O, forming carbonic acid (H2CO3) leading to ocean acidification. Between 1950 and 2020, the average pH of the ocean surface fell from approximately 8.15 to 8.05. The dissociation product of carbonic acid is the (HCO3), which harms marine ecosystems, particularly creatures with calcium carbonate shells or skeletons.
Carbon Dioxide is primarily introduced into the atmosphere through various natural and human activities:
- Natural Sources: CO2 is naturally released through processes like volcanic eruptions, respiration by plants and animals, and the decay of organic matter.
- Human Activities: The burning of fossil fuels (coal, oil, and natural gas) for energy production, transportation, and industrial processes is a major anthropogenic source of CO2 deforestation and land-use changes also release stored carbon into the atmosphere.
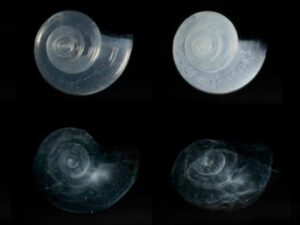
The dramatic increase of CO2 concentration in the atmosphere is human-caused. The fossil fuels we are burning right now contain carbon that plants pulled out of the atmosphere through photosynthesis over many millions of years, and we are now emitting that carbon to the atmosphere in a few hundred years. Annual CO2 emissions increased every decade starting from the middle of the 20th century, from close to 11 billion tons per year (1960s) to an estimated 36.6 billion tons in 2022.
Accurate monitoring and quantifying of CO2 levels in the atmosphere are crucial to assess progress in reducing greenhouse gas emissions and combatting climate change. Various techniques are employed to monitor and quantify CO2, each with its own strengths and weaknesses. These include:
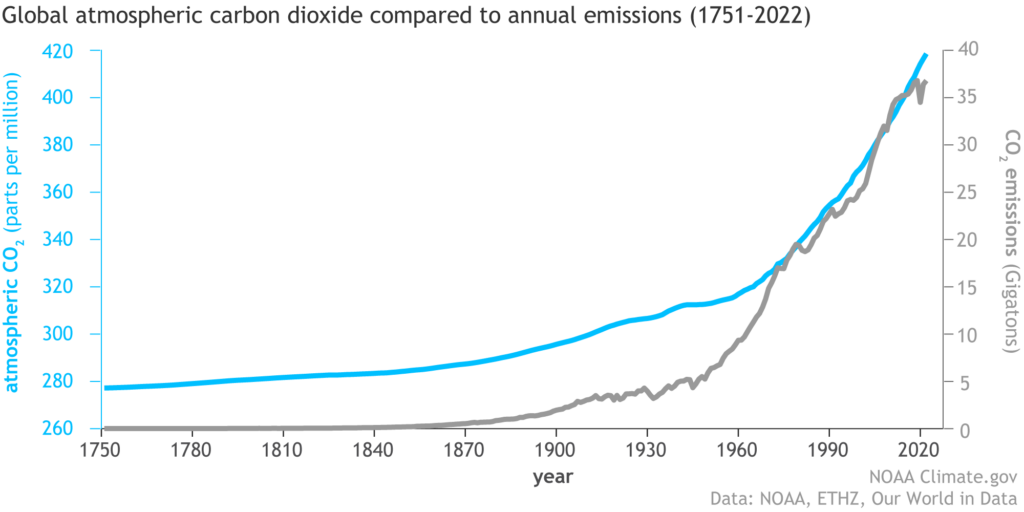
Non-Dispersive Infrared (NDIR) Sensors:
The most common sensors used to monitor CO2 are probably NDIR sensors. In general, NDIR uses broadband IR lamp sources and an optical filter (the non-dispersive element) in front of the detector to eliminate all light except the targeted wavelength. These sensors provide affordable and widely available, continuous, real-time measurements of CO2 levels. NDIR sensors typically have a long operational lifespan but are limited in sensitivity and require regular calibration to maintain accuracy. This simple approach is limited to a defined wavelength consequential gas species.
Semiconductor Gas Sensor:
When getting in contact with CO2, the semiconductor materials, typically metal oxides, react with it, affecting the electrical conductivity. The measured changes in the electrical resistance of the semiconductor material are proportional to the CO2 level. This approach presents a simple, low-priced and highly sensitive technique to monitor CO2. Its main disadvantages are the small linear measuring range and strong interferences from other gases.
Gas Chromatography:
Gas chromatography separates different gases accurately in a sample by passing them through a chromatographic column. CO2 molecules are then detected with high precision based on their interaction with a detector, usually a thermal conductivity detector (TCD) or a flame ionization detector (FID). This requires specialized equipment and expertise and it is typically used in a laboratory setting, limiting its application for real-time field monitoring.
Photoacoustic Spectroscopy:
Photoacoustic spectroscopy uses light to excite CO2 molecules at specific wavelengths. As CO2 molecules absorb the light, they undergo thermal expansion and generate pressure waves. The amplitude of these pressure waves is proportional to the CO2 concentration and can be detected. Photoacoustic spectroscopy offers high sensitivity (up to ppt) for trace gas measurements. As this technique is sensitive to environmental noise and influences, regular calibration and maintenance are essential and specialized equipment and expertise are required.
Electrochemical Methods:
The electrochemical reaction of an electrode inside the sensor with CO2 creates an electric signal. Depending on the type of these small-sized and long-living electrochemical sensors, this can be an electric current, potential difference, or resistance value. By comparing the signal to a reference potential obtained by a second electrode, the signal can be referred to the CO2 level leading to high sensitivities. The main drawbacks of these electrochemical sensors are the proneness to interfere with other gases and the vulnerability to environmental conditions.
Gravimetric/Absorption Methods:
Gravimetric/Absorption methods expose an absorbent (such as calcium chloride) to a well-defined volume of gas. CO2 is absorbed by the substance, increasing its weight. The change in weight can be measured extremely accurately to calculate the CO2 concentration. This complex technique is unsuitable for real-time measurements and requires maintenance and calibration.
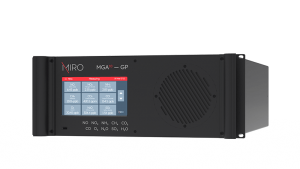
Here at MIRO Analytical, we use direct laser absorption spectroscopy in the mid-infrared region to monitor CO2 together with up to 9 other gases. Direct Laser Absorption Spectroscopy in the mid-infrared region stands out as an excellent technique for monitoring Carbon Dioxide (CO2) due to its high sensitivity and selectivity. It directly measures CO2 concentrations by detecting the rovibrational absorption lines, offering real-time monitoring capabilities and exceptional precision. This method is non-destructive, covers a wide dynamic range of CO2 concentrations, and is less susceptible to interference from other gases, making it suitable for diverse applications in research, atmospheric and environmental monitoring, and industry. Additionally, it enables isotopic analysis, making it valuable for tracing carbon sources in complex environmental systems.
Conclusion
Carbon Dioxide (CO2) is a molecule of immense significance influencing our climate, environment, and well-being. Through accurate monitoring and a better understanding of its sources and effects, we can take meaningful steps towards mitigating its impact and working towards a sustainable future. Join us next month as we continue our exploration of fascinating molecules shaping our world, and always remember: You cannot manage what you cannot measure!