Welcome to our latest installment of “Molecule of the Month,” where we delve into the intricate world of molecules shaping our environment and health. This month, our focus is on gaseous formaldehyde (CH2O or HCHO or H2CO), a compound of significant importance due to its widespread presence in both indoor and outdoor air. Join us as we explore the sources, impacts, and measurement techniques of gaseous formaldehyde.
Formaldehyde plays a crucial role in both indoor and outdoor environments, with several key implications:

Industrial Applications: Formaldehyde is used in various industrial processes, including the production of resins, plastics, and textiles. Its versatility and ability to crosslink with other molecules make it indispensable in manufacturing processes. However, industrial emissions contribute to outdoor air pollution and can impact human health and the environment.
Atmospheric Importance: In the atmosphere, gaseous formaldehyde is a key player in the oxidation of volatile organic compounds (VOCs) and hydroxyl radicals (OH). It serves as an intermediate in the formation of ozone and other secondary pollutants, contributing to air quality issues and regional haze. Understanding formaldehyde’s role in atmospheric chemistry is crucial for accurately modeling and predicting air pollution levels and their impacts on human health.
Indoor Air Quality (IAQ): Gaseous formaldehyde is a prominent indoor air pollutant, emitted from various sources such as building materials, furniture, and household products. Its presence in indoor air can lead to adverse health effects, including respiratory irritation, allergies, and other respiratory issues. Formaldehyde levels are particularly elevated in newly constructed or renovated buildings, highlighting the importance of monitoring and mitigating indoor formaldehyde concentrations.
Its sources can be devided into:
Industrial Emissions: Industrial activities, including chemical manufacturing, wood processing, combustion processes, fertilizers and pesticides are significant sources of gaseous formaldehyde emissions. These emissions contribute to outdoor air pollution, affecting air quality in surrounding areas and posing health risks to nearby communities.
Indoor Sources: Indoor sources of gaseous formaldehyde include building materials, furnishings, and consumer products such as paints, adhesives, and cleaning agents. Formaldehyde is also a by-product of combustion and certain natural processes. Therefore, activities like smoking, using unvented fuel-burning appliances, incense sticks or mosquito coils can significantly increase the levels of formaldehyde indoors.
Natural Sources: Formaldehyde is also emitted by natural processes such as forest fires, vegetation, and photochemical reactions in the atmosphere. While industrial and anthropogenic sources contribute significantly to formaldehyde levels, natural emissions also play a role in the atmospheric distribution of this compound.

IAQ is crucial because we spend approximately 90% of our lives indoors, and poor IAQ can lead to a range of health issues, including respiratory diseases, heart disease, cognitive deficits, and even cancer. Indoor air quality can vary stronger than outdoor. Individuals are often more directly exposed to emitting sources which leads to higher inhalation rates. Formaldehyde is a significant factor in indoor air quality due to its widespread use and presence in our daily lives. It is used extensively in the manufacturing of building materials and various household products which can lead to substantial concentrations indoors of up to 0.2 ppm compared to single digit ppb-levels outdoors. The emission rates of wooden furniture for instance depend on factors such as wood species, moisture content, outside temperature, and time of storage.
To estimate the release and accumulation of formaldehyde indoors with respect to emission rates, air exchange and exposure scenarios the European Reference Room was established. Assuming that the 31 m2 walls of the European Reference Room with 30 m3 volume are mad of medium density fiber board (MDF) with an emission rate of 80 µg/(m2h) the new European emission limit of 0.062 mg/m3 could be exceeded within 1 hour if no exchange with outdoor air occurs. This value highlights the importance of managing formaldehyde emissions to maintain good indoor air quality.
Accurate measurement of gaseous formaldehyde concentrations is essential for assessing human exposure levels and environmental impact. Despite other VOCs CH2O cannot be measured as part of the total VOCs by photoionization detectors PID. Several techniques are employed for formaldehyde detection:
Adsorbent Cartridge followed by Liquid Chromatography (HPLC):
This is a commonly used technique where airborne carbonyls react with 2,4-dinitrophenylhydrazine (2,4-DNPH) coated on an adsorbent cartridge. The hydrazone derivative is then separated and analyzed by HPLC with ultraviolet (UV) detection.
Gas Chromatography (GC):
Gas chromatography separates and quantifies formaldehyde in a sample based on its interaction with a chromatographic column. This method offers high sensitivity and specificity, making it suitable for laboratory analysis of air samples and industrial emissions.
Differential Optical Absorption Spectroscopy (DOAS):
DOAS is a specific type of absorption spectroscopy technique that separates the absorption cross sections into low and high frequency parts using specific numerical filtering methods. It is used to measure total column densities.
Fourier Transform Infrared Spectroscopy (FTIR):
Infrared spectroscopy detects gaseous formaldehyde based on vibrational absorption bands. This technique offers broadband, rapid and non-destructive analysis, making it suitable for both laboratory and field measurements of gas mixtures.
Chemical Ionization Mass Spectrometry (CIMS):
CIMS is a sensitive technique for formaldehyde detection, utilizing chemical ionization to ionize formaldehyde molecules, which are then analyzed using mass spectrometry. This method provides real-time measurements and high sensitivity for trace-level formaldehyde analysis.
Colorimetric Methods:
Colorimetric methods rely on the reaction between formaldehyde and specific reagents to produce a color change, which is then measured spectrophotometrically. While simple and cost-effective, colorimetric methods may lack the sensitivity required for trace-level analysis.
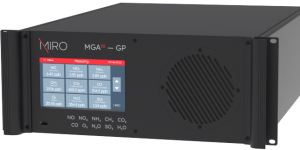
Here, at MIRO Analytical, we use direct laser absorption spectroscopy (LAS) in the mid-infrared region to monitor formaldehyde together with up to 9 other gases. This technique offers highest sensitivity, selectivity, and real-time monitoring capabilities, making it ideal to capture dynamic changes of CH2O at ppt levels for indoor and outdoor air quality monitoring. Its precision, wide dynamic range, and non-destructive nature contribute to its effectiveness in providing accurate and reliable data. Furthermore, LAS’ direct measurements of CH2O are less susceptible to interference from other gases.
Conclusion:
Gaseous formaldehyde presents complex challenges for indoor and outdoor air quality management, highlighting the need for effective monitoring and mitigation strategies. By understanding its sources, effects, and measurement techniques, we can take proactive steps to minimize exposure and ensure healthier living and working environments. Stay tuned for our next exploration into the captivating world of molecules shaping our planet and our lives!
Remember, you cannot manage what you cannot measure!