As we continue in our “Molecule of the Month” series, our focus turns to methane (CH4), a molecule with multifaceted impacts on Earth’s atmosphere and climate. Delve into the complexities of methane, exploring its diverse sources, sinks, and the array of techniques used for measurements.
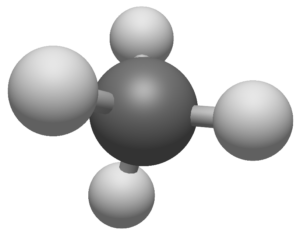
Methane, sometimes revered as natural gas, has great impact in the atmosphere. It is a potent greenhouse gas, with a global warming potential over 25 times that of carbon dioxide (CO2) over a 100-year period. Its ability to trap heat in the atmosphere contributes significantly to the greenhouse effect (20-30% of the anthropogenic greenhouse effect), influencing climate patterns and contributing to global warming. Ean indirectly impact air quality. Methane emissions often accompany other pollutants, leading to the formation of secondary pollutants such as ground-level ozone. These pollutants can have adverse effects on respiratory health and overall air quality. Methane release from thawing permafrost and destabilizing methane hydrates in the ocean pose potential feedback mechanisms. As the Earth warms, these previously stable reservoirs release additional methane, further amplifying the greenhouse effect.
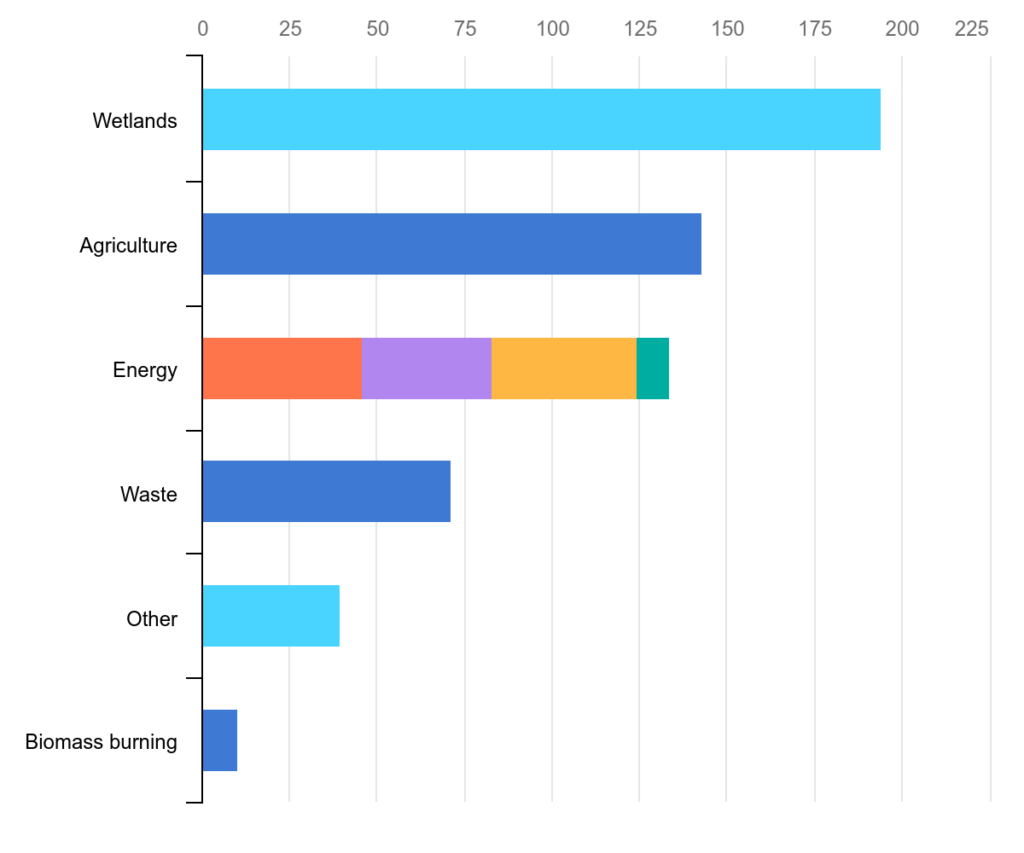
As illustrated in Figure 2 several natural and anthropogenic sources contribute to the increasing atmospheric methane level (the atmospheric methane concentration has more than doubled over the last 200 years). Methane is emitted naturally from various sources, including wetlands, termites, oceans, and geological processes. Natural emissions contribute a significant portion of the methane released into the atmosphere. Human activities play a substantial role in methane emissions. Fossil fuel extraction and processing, livestock digestion (enteric fermentation), rice cultivation, and the decay of organic waste in landfills are major anthropogenic sources of methane. An estimated 60% of today’s methane emissions are the result of human activities leading to a CH4 level in the atmosphere of >1920 ppb (January 2023).
On the other side, there are manifold sinks and a shorter lifespan of methane of about 10 years in the atmosphere compared to CO2. It is removed from the atmosphere through oxidation by hydroxyl radicals (OH) in the troposphere. Soil bacteria and other microbial processes also play a role in methane removal. Understanding these sink mechanisms is crucial for predicting methane’s atmospheric lifetime.
This rather short lifetime in the atmosphere and recent findings of satellite-based observation projects lead to a great interest in methane, lately. While methane was not even mentioned ones in the Paris agreement it became the most promising topic during the COP23. In contrast to CO2, methane emissions can be reduced rather easily, and the effect will be noticeable in a few years. Reducing human-caused emissions is one of the most cost-effective ways to avoid up to 0.3°C of global warming until 2040. Therefore, CH4 emissions should be reduced about 45% (180Mt/yr).
A first fast and cheap way is to avoid super-emitter events. Just one satellite-based project observed super-emitter events with a climate impact that is significantly larger than total greenhouse gas emissions of the Netherlands. In many cases, those leaks are easy to fix. Figure 3 shows for instance 12 super emitters close to each other in Turkmenistan with a flow rate of 50.400 kg/h.
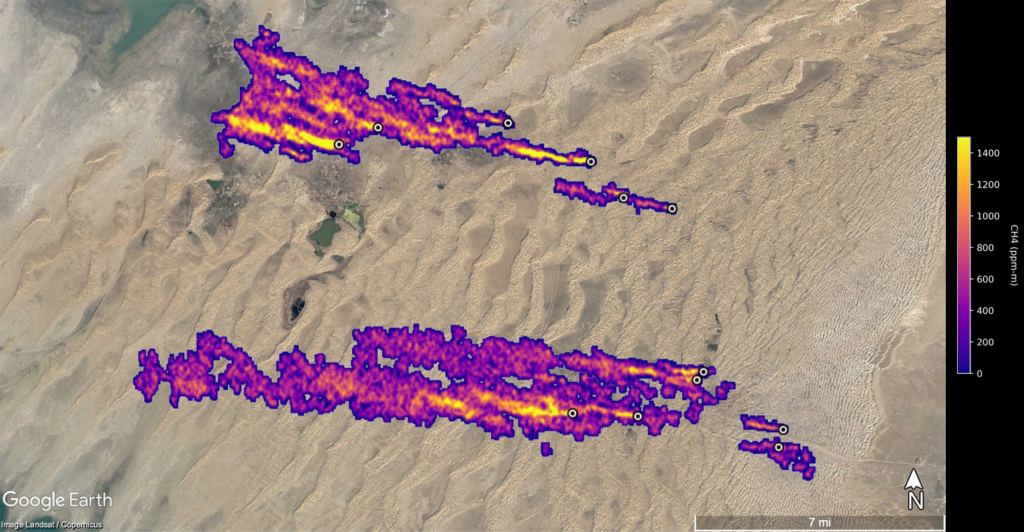
To support and verify such satellite-based monitoring, accurate ground-based measurement of methane concentrations are required to verify them. It is essential for understanding its sources, sinks, and overall impact on the environment. The most common techniques are:
Semiconducting Metal Oxide Sensors:
These sensors detect methane by measuring changes in resistance of a metal oxide when exposed to methane gas. They are known for their durability and low cost, making them suitable for portable devices. The main disadvantages are their lower selectivity and the need for high operating temperatures.
Electrochemical Sensors:
Electrochemical sensors work by measuring the current generated by the electrochemical reaction of methane on an electrode surface. They offer the advantage of being highly sensitive and capable of continuous monitoring. However, their performance can be affected by humidity, temperature, and the presence of other gases, requiring regular calibration and maintenance.
Cavity Ring-Down Spectroscopy (CRDS):
CRDS measures methane concentrations by analyzing the decay time of light within an optical cavity. It offers high sensitivity and precision, making it effective for both laboratory and field measurements. The main drawback is the high cost of equipment and the need for technical expertise.
Proton Transfer Reaction Mass Spectrometry (PTR-MS):
PTR-MS detects methane through the mass-to-charge ratio of ions formed during proton transfer reactions. It provides real-time, sensitive measurements ideal for atmospheric monitoring. However, its complexity and cost can be limiting factors.
Gas Chromatography:
This laboratory-based method separates methane from other gases in a sample, providing detailed concentration and isotopic composition. Its advantage lies in its accuracy and ability to analyze complex mixtures. However, it requires sophisticated equipment and skilled operation, limiting its use to laboratory settings.
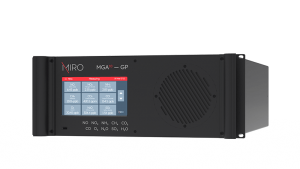
Here, at MIRO Analytical, we use direct laser absorption spectroscopy (LAS) in the mid-infrared region to monitor methane together with up to 9 other gases. This technique offers high sensitivity, selectivity, and real-time monitoring capabilities, making it ideal for capturing dynamic changes in CH4 levels as it is done in Eddy covariance measurements. Its precision, wide dynamic range, and non-destructive nature contribute to its effectiveness in providing accurate and reliable data. LAS direct measurements of CH4 are less susceptible to interference from other gases, making it suitable for diverse applications in research, atmospheric and environmental monitoring, and industry.
Conclusion:
Methane, with its intricate role in the greenhouse effect, air quality, and global climate, demands comprehensive understanding and precise measurement. From natural sources to human caused super-emitters, and from atmospheric removal processes to potential feedback mechanisms, the study of methane continues to be a crucial aspect of environmental science.
As we wrap up our exploration of molecules shaping our world this year, let’s reflect on the importance of advancing our knowledge based on precise measurements to fight climate change more efficiently. Join us next year as we embark on new journeys, unraveling the mysteries of molecules and their profound influence on our planet. Always remember: You cannot manage what you cannot measure!