Welcome to this month’s installment of “Molecule of the Month,” where we explore the significant yet often overlooked molecules that impact our environment and health. This month, our focus is on carbon monoxide (CO), a colorless, odorless gas with far-reaching implications for human health and atmospheric chemistry. Join us as we delve into the sources, effects, and measurement techniques for this insidious gas.

Carbon monoxide is a colorless, odorless, and tasteless gas that is both a common environmental pollutant and a critical component in atmospheric chemistry. Here’s why CO is significant:
Health Impact: CO is often referred to as the “silent killer” due to its high toxicity and the difficulty in detecting it without specialized equipment. When inhaled, CO binds with hemoglobin in the blood, reducing its ability to transport oxygen throughout the body. This can lead to symptoms ranging from headaches and dizziness to severe hypoxia and even death in high concentrations. Vulnerable populations, such as individuals with cardiovascular conditions, children, and the elderly, are particularly at risk. Therefore, the WHO recommends the indoor air level for CO to be below an average of 80 ppm for a short term exposure of max 15 min, and below 5.6 ppm for a 24 h period.
Environmental Impact: In the atmosphere, CO plays a role in the formation of ground-level ozone, a major component of smog. It also reacts with other atmospheric components, contributing to the formation of carbon dioxide (CO2) and methane (CH4), both potent greenhouse gases that drive climate change.
Atmospheric Chemistry: CO is a key player in atmospheric chemistry, particularly in the oxidation of methane and other hydrocarbons. It acts as an important sink for hydroxyl radicals (OH), influencing the overall oxidative capacity of the atmosphere. This affects the lifetimes of many atmospheric pollutants and greenhouse gases, making CO a critical molecule in the study of air quality and climate dynamics.
Sources and Effects:
Anthropogenic Sources: The primary sources of CO from human activities include incomplete combustion of fossil fuels in vehicles, industrial processes, residential heating, and cooking. These sources contribute significantly to urban air pollution and can pose serious health risks in densely populated areas.
Natural Sources: Natural sources of CO include wildfires, volcanic eruptions, and the oxidation of methane and other organic compounds emitted by plants and soils. While these sources contribute to the global CO burden, their impact is generally less concentrated compared to anthropogenic sources.
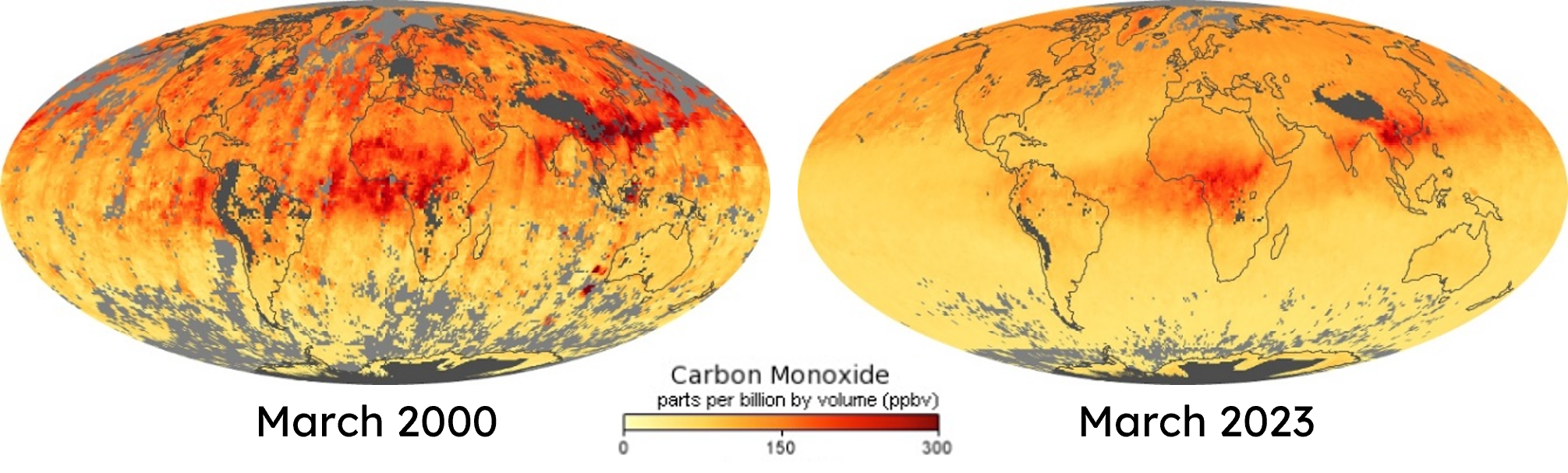
Since 2000, a declining trend in the global CO budget has been observed. This is probably mainly driven by three measures: (I) the reductions in the transport sector, the anthropogenic emissions in the US and Europe, and (II) reductions obtained in the industry and residential sectors in China, as well as (III) by reduced biomass burning emissions globally, especially in equatorial Africa and South America.
Not a greenhouse gas, but strong impact to global warming:
CO, while not a greenhouse gas itself, significantly impacts the atmospheric concentration of other greenhouse gases, particularly methane (CH4). This is due to a series of chemical reactions involving the hydroxyl radical (OH). In the atmosphere, CO reacts with hydroxyl radicals to form carbon dioxide (CO2) and water (H2O).
This reaction consumes hydroxyl radicals which otherwise could active methane, a potent greenhouse gas by reducing it to its radical:
However, if hydroxyl radicals are being consumed by reactions with CO, there are fewer hydroxyl radicals available to react with methane. As a result, the rate at which methane is removed from the atmosphere decreases, effectively increasing the lifespan and concentration of methane in the atmosphere.
Since methane is a much more potent greenhouse gas than carbon dioxide, this can lead to an amplified greenhouse effect, contributing to global warming. Therefore, while CO is not a greenhouse gas, its presence in the atmosphere can indirectly increase the concentration of other greenhouse gases, thereby influencing global warming.
Accurately measuring CO concentrations is essential for assessing its impact on air quality and human health. Various techniques are employed for CO detection:
Cavity Ring-Down Spectroscopy (CRDS): This is an optical technique where light is trapped in a cavity and its decay or ‘ring-down’ is measured. The presence of CO alters the ring-down time, which can be used to measure its concentration. While this method offers high sensitivity, precision, and accuracy, it may experience potential interference from water vapor on the CO amount fraction reported by near-IR CRDS instruments.
Off-Axis Integrated Cavity Output Spectroscopy (OA-ICOS): This is a variant of CRDS where light does not need to be aligned along the cavity axis, making it more robust to alignment issues. OA-ICOS provides high sensitivity and is more robust to alignment issues compared to CRDS. Similar to CRDS, it can potentially experience interference from water vapor.
Fourier Transform Infrared Spectroscopy (FTIR): This technique measures the absorption of infrared light by CO molecules. The absorption pattern is unique to CO and can be used to determine its concentration. FTIR can measure multiple gases simultaneously and has a wide spectral coverage, but it lacks sensitivity.
Non-Dispersive Infrared Spectroscopy (NDIR): This method measures the absorption of infrared light by CO. The amount of light absorbed is directly proportional to the concentration of CO. NDIR is simple, robust, and cost-effective but Limited to gases that absorb in the narrow spectral range and can be affected by other gases that absorb at the same wavelength.
Gas Chromatography (GC): This technique separates the components of a gas sample and measures the concentration of each component, including CO. GC can measure multiple gases simultaneously but requires pre-treatment of samples and is more complex and time-consuming than the other methods.
Mass Spectrometry (MS): This method ionizes gas molecules and separates them based on their mass-to-charge ratio. This is a highly sensitive technique and can measure multiple gases simultaneously, but it is expensive, complex, and requires skilled operators.

Here, at MIRO Analytical, we use direct laser absorption spectroscopy (LAS) in the mid-infrared region to monitor CO together with up to 9 other gases. This technique offers highest sensitivity, selectivity, and real-time monitoring capabilities, making it ideal for ambient CO measurements. As a robust multi-compound gas analyzer with high time resolution (up to10 Hz), MIRO´s MGA is ideal for measurements of CO, CO2, CH4, O3, NO and NO2 which are being involved in the complex atmospheric chemistry of CO. LAS’ precision, wide dynamic range, and non-destructive nature contribute to its effectiveness in providing accurate and reliable data. Furthermore, it’s direct measurements of CO are less susceptible to interference from other gases.
Carbon monoxide is a silent yet significant player in both environmental pollution and atmospheric chemistry. Understanding its sources, effects, and measurement techniques is crucial for mitigating its impact on human health and the environment. By monitoring and controlling CO emissions, we can improve air quality and protect public health, contributing to a cleaner and safer atmosphere. Stay tuned for our next exploration into the fascinating world of molecules shaping our planet and our lives! And keep in mind, you cannot manage what you cannot measure!